Photosynthesis: Main Concepts
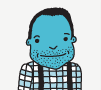
Photosynthesis: Main Concepts
Modes of nutrition
Photosynthesis is the process in which light energy from the sun is transformed into chemical energy that is later used to synthesise large organic molecules from simple inorganic molecules. This means the way in which organisms obtain the energy the need can be divided into two categories. Autotrophs(meaning ‘self- feeding’) can build up complex organic molecules like proteins, carbohydrates and lipids from simple inorganic molecules such as carbon dioxide and water. Photoautrophs absorb light and use this as their energy source and this would include plants, bacteria and algae. Heterotrophs meaning ‘other- feeding’) obtain their energy by the breakdown of large organic molecules into smaller ones. Animals, fungi and some bacteria are heterotrophs.
Essays on this topic:
Investigate the factors which affect photosynthesis.
Compare and contrast the structure and function of Chloroplasts and Mitochondria
The Chloroplast
In higher plants, photosynthesis takes place in organelles called chloroplasts. These organelles are adapted for their role of harvesting light energy, and carrying out both the light-independent and light-dependent stages of photosynthesis. A double membrane surrounds the chloroplast. The inner membrane is folded to increase the available surface area and is selectively permeable. Inside the chloroplast is a complex system of membranes called lamellae (meaning small plates). In places these form fluid-filled, disc-like structures called thylakoids. The light absorbing pigments, enzymes and electron carriers needed for the light-dependent stage are found in the thylakoids. Thylakoids are built up into little stacks called grana (singular granum), and the enzymes necessary to make ATP (ATP synthase) are attached to them. The stroma is a fluid–filled matrix that contains the enzymes necessary for the light-independent stage of photosynthesis. Chloroplasts contain both a loop of DNA and ribosomes, which means they can make some of the proteins they need to use during photosynthesis.
Example essays:
Compare and contrast the structure and function of Chloroplasts and mitochondria '10^2'
Light harvesting
The primary, light absorbing pigments, both forms of chlorophyll a
, and other light absorbing pigments, called accessory pigments, are arranged into little clusters called photosystems on the surface of the grana. There are two primary pigments, which are both forms of chlorophyll a
, and they each absorb photons of light of slightly different wavelengths. Photosystem I has a chlorophyll a
molecule at its centre that has a maximum absorption at 700nm. Photosystem II has a chlorophyll a
molecule with a maximum absorption at 680nm.The accessory pigments include other forms of chlorophyll a
, chlorophyll b
, and carotenoids. There may be a collection of several hundred different pigment molecules in a photosystem. Chlorophyll molecules absorb light mainly in the red and blue regions of the spectrum whereas carotenoids absorb mainly in the blue- violet region. Combining pigments with different absorption peaks can absorb a greater proportion of the available light energy.
These pigments absorb photons of light and create a wave of excitation energy, which is transferred through the cluster of pigments until it reaches a particular chlorophyll molecule at the centre called the reaction centre.
The light-dependent reaction
The light-dependent reactions take place within the thylakoid membranes of the chloroplast and involve two key chemical reactions. These are the synthesis of ATP from ADP and inorganic phosphate called photophosphorylation and photolysis. A phosphorylation reaction adds a phosphate group to another molecule and this particular reaction is called photophosphorylation since the energy needed for the reaction to occur, came originally from the photons absorbed by the pigments in the photosystem. Photophosphorylation can be cyclic or non-cyclic depending on the pattern of electron flow. Photolysis is the splitting of a water molecule which produces electrons (e-), hydrogen ions (H+), (also called protons), and oxygen.
Essay examples:
Investigation to find out how light intensity effects the rate of photosynthesis
Cyclic photophosphorylation
Cyclic photophosphorylation only involves photosystem I (also known as P700) forms ATP. The chlorophyll a
molecule in the reaction centre is sensitive to light (photosensitive) and when it absorbs light energy it causes an electron to be excited to a higher energy level. The electron gains so much energy it leaves the chlorophyll molecule and is picked up by another molecule called an electron acceptor or carrier. The electron is then passed back to the chlorophyll a
along a chain of other electron carriers. As it is passed along the chain, the energy it contained is released and is used to make an ATP molecule from ADP and phosphate. The ATP that is made can then be used in the light-independent reactions. The electron, now at a lower energy level, returns to the chlorophyll a
molecule in photosystem I.
Non-cyclic photophosphorylation
This involves both photosystem I and II. Light is absorbed by photosystem II and this boosts electrons to a higher level, where they are picked up and then passed along a chain of carriers to photosystem I. The energy released is used to make ATP from ADP and inorganic phosphate. The electrons are accepted by the chlorophyll a
in photosystem I. Photolysis takes place. This is the splitting of water, catalysed by an enzyme found within the photosystem. H
2
0→2H
+
+2e
−
+1
2
0
2
The electrons released replace those lost by photosystem II. The oxygen can be used in respiration, or if in excess, is released as a waste product. The Hydrogen ions will be used later by photosystem I. Light energy absorbed by photosystem I boosts the electrons to an even higher energy level where they are picked up by another chain of electron carriers. These electrons are used, together with the hydrogen ions from photolysis to reduce a molecule called NADP. 2H
+
+2e
−
+NADP→reducedNADP
Some useful essays:
The Light-independent reaction
The enzymes for the light-independent stage are found in the stroma of the chloroplast. In this process carbon dioxide is reduced to form simple sugars in a process known as the Calvin cycle. In the first step a 5-Carbon compound called ribulose bisphosphate (RuBP) combines with a molecule of carbon dioxide. This process is called carbon fixation. It is a carboxylation reaction and is carried out by an important enzyme called rubisco (its full name is ribulose bisphosphate carboxylase). It forms an unstable 6-Carbon compound, which immediately splits apart into two molecules of a 3- Carbon compound called glycerate 3- phosphate (GP). The reduced NADP and the energy from ATP are used in the next step to reduce GP to a 3-Carbon sugar known as triose phosphate. Some of the triose phosphate molecules (one sixth of the molecules formed) is used to make carbohydrates such as glucose and the rest (five sixths) is used to regenerate RuBP, so that the cycle can continue. ATP energy is needed for the conversion of triose phosphate to ribulose bisphosphate.
The end products of the light- independent stage are organic molecules. GP can be made into glycerol and TP can be made, for example into hexose sugars, starch and cellulose as well as amino acids and proteins. A source of nitrogen atoms is needed to make amino acids and then proteins. All of the many biochemical molecules found in plants ultimately are made from these simple products of photosynthesis.
Useful Essays:
Biology Lab Report. Does light intensity affect the rate of photosynthesis?
Investigation to find out how light intensity effects the rate of photosynthesis
Investigating factors that affect the rate of photosynthesis
The chief external factors affecting the rate of photosynthesis are the light intensity, temperature and carbon dioxide concentration. It should also be noted that water availability, chlorophyll concentration, oxygen concentration and environmental atmospheric pollutants such as ozone and sulfur dioxide could also affect the rate of photosynthesis.
If an investigation is undertaken to examine the effect of light on photosynthesis then this term must be defined since it could refer to light intensity, light wavelength or the duration of exposure to light (photoperiod).
Example essays:
Investigating Factors Affecting the Rate of Photosynthesis.
Rate of Reaction in Photosynthesis
Measuring the rate of oxygen production
Measuring the rate at which oxygen is released seems to be an obvious way to investigate the effect of varying key variables on the rate of photosynthesis. The problem is that plants carry out respiration as well as photosynthesis and this process uses oxygen and releases carbon dioxide. Most experiments can be used to measure the net rate of photosynthesis under certain conditions as long as this factor is recognized.
A convenient way to measure oxygen release is to use an aquatic plant and count the number of bubbles released from the stem in a fixed time. However, this method is not reliable since the bubbles vary in size and the assumption is made that the bubbles are pure oxygen, when they may contain carbon dioxide and possibly nitrogen from the water. The bubbles of gas should be collected in a fixed period of time and their volume measured. The bubbles of gas can be collected in a special piece of apparatus called a photosynthometer. It is possible to analyse the gas collected for both oxygen and carbon dioxide concentrations, using a technique that uses a capillary tube containing some of the collected gas and the chemicals potassium hydroxide and pyrogallol, which react with carbon dioxide and oxygen respectively.
Essays on this topic:
Demonstrating the production of oxygen during photosynthesis
Measuring the rate of carbon dioxide uptake
Hydrogen carbonate indicator can be used to show the changes in pH that take place as carbon dioxide is removed from a solution. If the indicator is equilibrated with normal atmospheric air then it has a red/orange colour. It becomes yellow at a greater carbon dioxide concentration and a deeper red and finally purple if carbon dioxide is used. It is non-toxic and can be used with aquatic plants in solution or with algae that have been immobilised into jelly-like beads made from sodium alginate. Changes in colour can be measured by measuring the absorbance of the indicator solution in a colorimeter using the appropriate filter (550nm). A range of pH buffers could be used to produce arrange of colours for comparison purposes if a colorimeter is not available.
More essays:
Effects of temperature and carbon dioxide on photosynthetic rate in Elodea.
The Effect of Carbon Dioxide Concentration On The Rate of Photosynthesis.